Adjustable muscular damping to increase 'morphological computation' during locomotion with legs
The neuromuscular control system of animals (and humans) produces seemingly effortless robust and energy-efficient locomotion, even on uneven surfaces. This is often still a challenge for robotic systems. In the biological system, the viscoelastic properties of the muscle and its tendon play an essential role in this regard: the optimal coordination of energy generation, energy dissipation, and energy storage. Especially in unknown ground conditions, where the kinetic energy of the system varies with each ground contact, the viscoelastic properties of the muscle generate the initial response to disturbances (e.g., due to ground unevenness). Thus, despite neural delays (of up to 40% of the duration of a stance phase), the muscle contributes to robust and agile locomotion through so-called "morphological computation." In detail, the viscoelastic properties of the muscle strongly depend on muscle activation and are thus neuronally adjustable. In particular, the contribution of damping has not been systematically investigated. Thus, the core research question of this project is: How can biological and robotic systems exploit the adjustable damping properties of muscle for efficient and robust locomotion? Specifically, the following hypotheses will be addressed: 1) "Morphological computation" can be enhanced by mechanical damping. 2) By adjusting damping, it is possible to extend the operating range of serial elastic actuators to produce a wider range of velocities and accelerations. 3) Adjustable damping allows the trade-off between "morphological computation", energy efficiency, robustness and variability of motion to be tailored to the requirements of the environment.
These hypotheses will be tested through the development and close interaction of three modeling approaches: a) Development of a neuromuscular biomechanical computer model that incorporates new insights and hypotheses, particularly on viscous muscle damping. b) Development of a biorobotic leg model that incorporates muscle-inspired passive but tunable mechanical damping. c) Development of a muscle fiber model that, based on experimentally determined viscoelastic muscle properties, allows realistic predictions of eccentric force response during ground contact as well as energy efficiency during cyclic locomotion. This interdisciplinary research project will therefore combine computer modeling, robot design, and muscle physiology approaches to investigate the fundamental contribution of "morphological computation" to robust locomotion. In particular, the exploitation of muscular damping by low-level control will be quantified for the first time in terms of "morphological computation".
Figure 1: Nerve conduction delays due to limited nerve conduction velocities in organic tissue [57] 2 present a significant hazard in legged locomotion, but local neuromuscular strategies 6 provide an alternative means of timely and tunable force and power production. Muscles like the indicated knee extensor keep the leg extended during locomotion (muscle length Lmuscle), and produce the required muscle forces (Fmuscle), with appropriate amount and timing. Neuromuscular control 1 plays a major role producing active muscle forces, especially during unperturbed locomotion. Sensory information, i.e., from foot touchdown, travels via nerves bundles 2 to the spinal cord, but with significant time delays in the range of 40 ms [56] and more. Hence, the locomotion control system can become ’sensor blind’ due to conduction delays, for half a stance phase, and longer. During step-down perturbations 3 additional energy 4 is inserted into the system, and must be countered through additional muscle forces, timely produced. Local neuromuscular strategies 6 producing tunable, viscous damping forces could act instantaneously and
adaptively. Such 6 strategies could also be robust to uncontrolled and harsh impacts of the foot after perturbations 5 , in comparison to sensor-based strategies.
Joint publications:
- Araz M, Weidner S, Izzi F, Badri-Spröwitz A, Siebert T and Haeufle DFB (2023). Muscle preflex response to perturbations in locomotion: In vitro experiments and simulations with realistic boundary conditions. Front. Bioeng. Biotechnol. 11:1150170. [link]
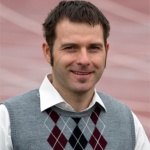
Tobias Siebert
Prof. Dr.Institutsdirektor
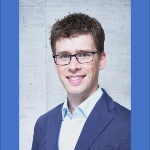
Daniel Häufle
Dr.Kooperationspartner Universität Tübingen
[Bild: Daniel Häufle]
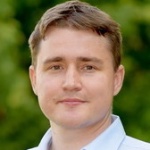
Alexander Badri-Sprowitz
Dr. Sc.Kooperationspartner MPI
[Bild: Alexander Badri-Spröwitz]